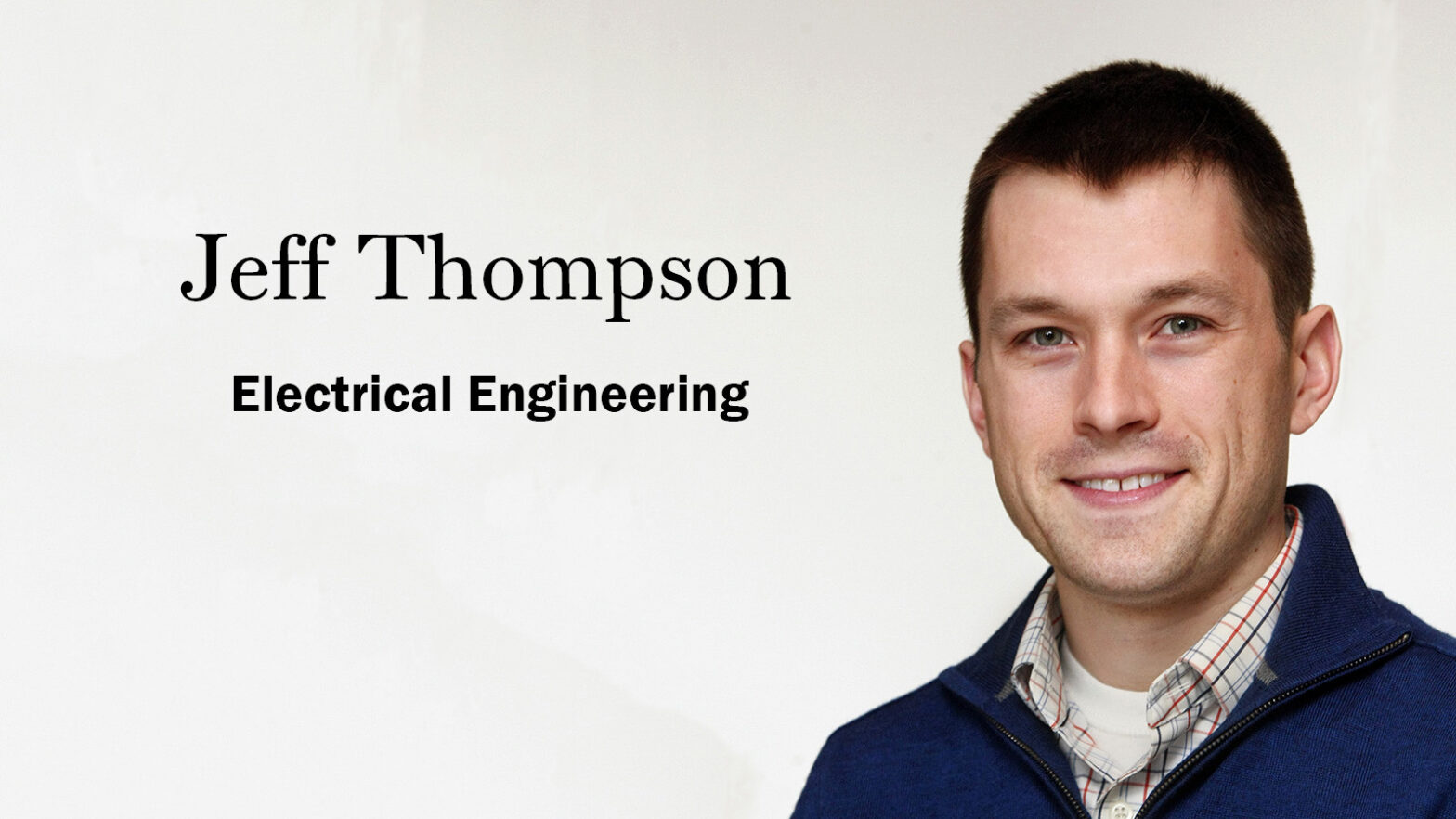
Thompson ‘wrangles’ ornery atoms for quantum technologies
By
on
The room-filling machinery looks diabolical, clicking and whirring with wires running all over the place. Perched at a computer terminal linking him to the mysterious instrument is Jeff Thompson, a new faculty member in Princeton’s Department of Electrical Engineering.
Thompson directs half a dozen lasers within this giant apparatus to bear down upon a single atom inside an airless chamber. The lasers trap the lone particle of matter in space, causing the atom’s motion to nearly cease and thus cooling it to within a whisker of absolute zero. In these exotic conditions, the bizarre, quantum mechanics of individual atoms-hidden in everyday circumstances-surge to the fore.
“I call it ‘atom wrangling’,” said Thompson. “It’s pretty amazing that you can do this. You have one atom just floating there, and you can pin down its position and control it with very high precision.”
Thompson and his colleagues aim to wrangle many such atoms into someday serving as the workhorses for quantum computers, which can perform certain tasks phenomenally faster than today’s conventional supercomputers. Other applications include building intrinsically secure quantum communication networks as well as miniscule, energy-efficient sensors.
“Quantum mechanics – this set of laws that underlies the universe and which we’ve known about for a hundred years – offers us a lot of technological opportunities we haven’t taken advantage of or even realized yet,” said Thompson.
The groundbreaking technologies Thompson is pursuing will rely on both retaining and directing the quantum mechanical behaviors of atoms and groups of atoms. Bucking all our conceptions of ordinary reality, in the quantum mechanical realm, atoms can quite literally be in two places at once. Upping the weirdness ante, two atoms’ properties can also become “entangled,” such that measuring one particle at any distance-from millimeters to miles-from its counterpart particle in effect instantaneously determines the second particle’s properties.
Counterintuitive as these quantum mechanical laws sound, physicists actually have a quite thorough understanding of them, at least when it comes to the theoretical actions of single atoms. Extrapolating these descriptions to real-life, physical systems of many billions of interacting atoms, however, remains a daunting and stimulating challenge for Thompson and his colleagues in the field.
“From a physics perspective, it’s interesting because we know pretty much all the laws of quantum mechanics as they relate to what we’re doing-there are no new forces we’re going to discover,” said Thompson. “But what happens when you put all these atoms together is that things get really complicated, with phenomena cropping up that you could not predict based on the properties of the individual atoms.”
Trapping increasingly large sets of atoms with lasers to probe and further understand their quantum peculiarities is one way to skin the quantum mechanical cat. Another method Thompson employs is the study of impurities in otherwise perfect crystals. Specifically, he focuses on so-called rare earth ions. These are electrically charged atoms of certain elements that have unique chemical properties. When ensconced in a crystal, rare earth ions can intriguingly behave like those isolated atoms Thompson lassoes with lasers. “Working with these rare earth ions is a new frontier,” said Thompson.
One key advantage with solid-state materials, like crystals, is the obviating of room-sized apparatuses to stabilize free-wheeling atoms. Secondly, the atom density in crystals is far higher than in groups of atoms herded by lasers. The atoms’ proximity allows them to interact, so the quantum state of one can influence the quantum state of another. “We want to be able to have this interaction happen in a clean and controllable way,” said Thompson.
Using light, Thompson works on precisely locating rare earth ions within crystals and measuring their quantum mechanical states. The light also doubles as a tool for altering that quantum state. Ions that absorb the light re-emit it after a short period of time, which yields information about what changed during the process.
Manipulation of this delicate input-output in crystals doped with rare earth ions could form the material basis of a quantum memory system, a main goal of Thompson’s work. In such a system, quantum information contained in individual atoms would act like the bits-the ones and zeroes-of the hard drive memories in conventional computers and telecommunications networks.
In a quantum telecom setup, hackers could not access the transmitted or stored quantum information without altering it, thereby alerting its sender and receiver of a break-in, so any communiqués would be inherently secure. So-called repeater stations, equipped with quantum memory like that pursued by Thompson, could preserve this fragile quantum information and help relay it over long distances via fiber optic cables.
Thompson has covered some long distances himself in his academic career. He grew up in Houston, Texas, then attended Yale for an undergraduate degree in physics. Thompson then decamped for atomic physics work in Germany. His research there led him back stateside to Harvard for his doctorate, the Massachusetts Institute of Technology as a postdoctoral fellow, and onward to Princeton.
Throughout his career, Thompson has yearned to take furtive quantum mechanics up to a level that is obvious to us normally oblivious humans.
“I’m drawn to making quantum mechanical effects more obvious somehow and in such a way that you can really notice their consequences,” said Thompson. “I’ve been through several different scientific fields and that’s the thread that ties it all together.”