For decades, scientists have puzzled over how a symmetrical ball of cells becomes an embryo, with the beginnings of a head and tail. Now Princeton engineers have discovered that this transformation from perfect symmetry to different shapes and functions can be predicted much earlier than previously thought. They developed a tool to trace chemical messages that spur the changes in mammals, with implications for better understanding developmental disorders.
The researchers started with a basic question: What’s the earliest chemical signal we can find in this ball of cells that predicts whether a cell ends up on the “head” side or the “tail” side of the embryo?
One of the challenges is that, for mammals, these cells are inside a mother. “It’s really hard to study them because you can’t watch them do stuff in front of you on the microscope,” said principal investigator Jared Toettcher, associate professor of molecular biology and the Omenn-Darling Bioengineering Institute. He and Lewis-Sigler Scholar Harry McNamara turned to stem cell models that mimic certain functions of a mammal embryo.
McNamara then engineered genes into a circuit that could sense, record and track when the cells receive their key chemical signals, including one called Wnt (pronounced “wint”). This Wnt signal is associated with many developmental functions in mammals; in embryos they are known for their role in this “head and tail” question. With this circuit in the stem cells and no added external chemical signals in the model, the researchers made two important observations.
First, they found two other signals that also play a role in the head and tail question, and importantly, they detected them earlier than the Wnt signal. This means the change from symmetrical ball to asymmetrical embryo-like structure in the stem cell model can be traced to much earlier changes in cellular states. “Much earlier than anyone would predict,” said Toettcher.
Second, the team observed how the tail cells moved and organized themselves. It turns out that the cells can control how much or how little they can physically stick to each other. The tail cells will stick to their fellow tail cells and not to other cells. As the ball of stem cells grows, the tail cells eventually all gather in a single location. This phenomenon is known as cell sorting. (Notably, the mechanism of how cells stick together and move about was proposed by the late Princeton biologist Malcolm Steinberg.)
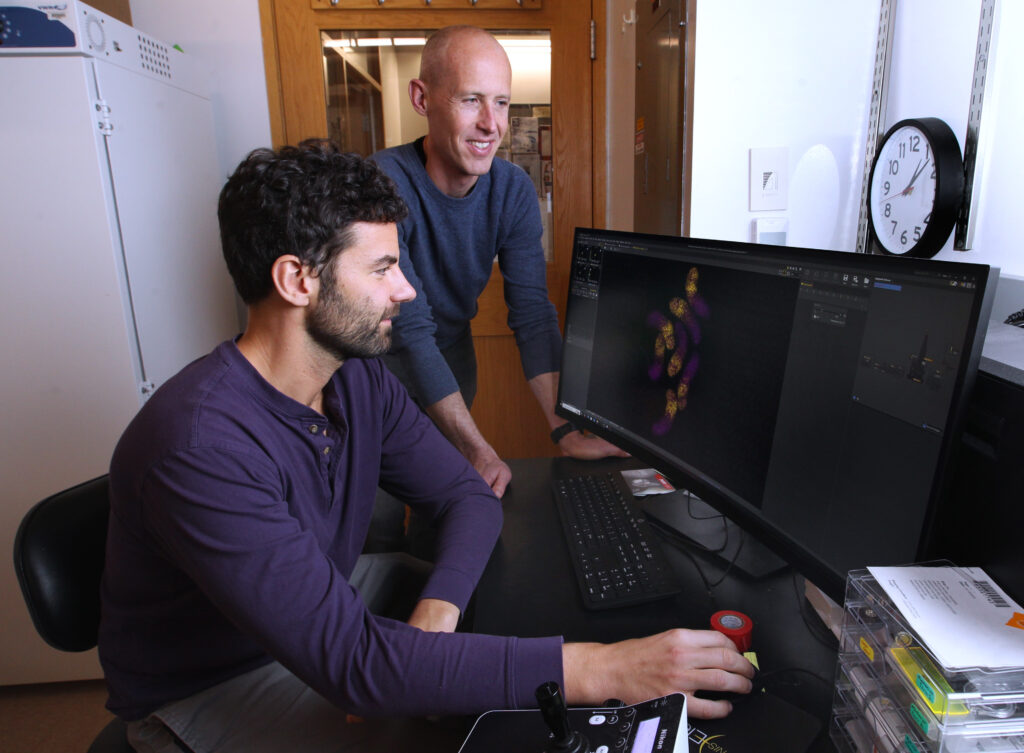
Their observations point to cell sorting as an explanation for the head and tail question, and perhaps a more minor role for external signals than previously thought.
Toettcher said the findings point in exciting new directions for understanding mammalian development, but cautioned that these findings are currently limited to the embryo-like stem cell model. “It is not at all obvious that this is exactly the same process that happens in the embryo itself,” said Toettcher.
That said, the tool they built could be used to identify other internal cues that drive other important developmental processes. That’s especially true when it comes to using stem cell models to study embryos — an approach that is still new in the field. “There is a ton of potential in these stem cell models,” said McNamara, for both basic science and applications that could improve human health.
The paper, “Recording morphogen signals reveals mechanisms underlying gastruloid symmetry breaking,” was published Oct. 2 in Nature Cell Biology. In addition to Toettcher and McNamara, the authors included Sabrina C. Solley, Britt Adamson and Michelle Chan of Princeton University. Support for the project was provided by the Lewis-Sigler Scholars program, the National Science Foundation, the National Institutes of Health and the Vallee Foundation.