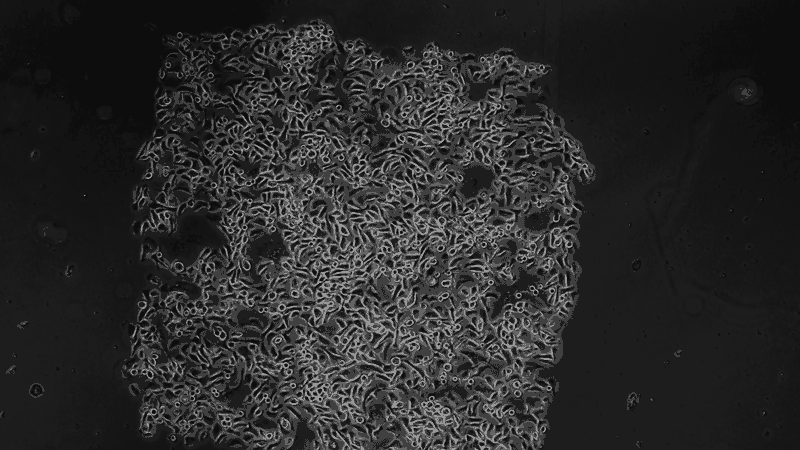
Researchers use electric fields to herd cells like flocks of sheep
By
on
Scientists have long known that naturally occurring electrochemical signals within the body can influence the migration, growth and development of cells – a phenomenon known as electrotaxis. These behaviors are not nearly as well understood as chemotaxis, in which cells respond to chemical concentration differences. One barrier has been a lack of accessible tools to rigorously examine cells’ responses to electric fields.
The new system, assembled from inexpensive and readily available parts, enables researchers to manipulate and measure cultured cells’ movements in a reliable and repeatable way. In a paper published June 24 in Cell Systems, the Princeton team described the assembly and preliminary studies using the device, which they call SCHEEPDOG, for Spatiotemporal Cellular HErding with Electrochemical Potentials to Dynamically Orient Galvanotaxis. (Galvanotaxis is another term for electrotaxis.)
Previous systems for studying cells’ responses to electric fields have been “either bespoke and handmade, with issues of reproducibility, or requiring fabrication facilities that make them expensive and inaccessible to many labs,” said co-lead author Tom Zajdel, a postdoctoral research fellow in mechanical and aerospace engineering. “We wanted to use rapid prototyping methods to make a well-defined device that you could just clamp onto your petri dish.”
While there is a long history of work on electrotaxis, said Zajdel, the phenomenon is not well understood. Evidence shows, for example, that reversing the direction of a natural electric field can inhibit wound healing in animal models, while amplifying the existing field might improve healing.
“There are a lot of unknowns about how individual cells detect such fields,” said senior author Daniel Cohen, an assistant professor of mechanical and aerospace engineering. “But the beauty of crowd dynamics is that even if you don’t understand everything about the individuals, you can still engineer behaviors at the group level to achieve practical results.”
The SCHEEPDOG device contains two pairs of electrodes that are used to generate electric fields along horizontal and vertical axes, as well as recording probes to measure voltage and integrated materials to separate the cells from chemical byproducts of the electrodes. The voltage level is similar to that of an AA battery concentrated over the centimeter-wide chamber containing the cells.
“It’s kind of like an Etch A Sketch,” said Zajdel, referring to the classic drawing toy in which lines can be created in any direction by turning two control knobs. “We’ve got the horizontal and the vertical knobs, and we can get the cells to trace out arbitrary trajectories in the whole 2-D space just by using those two knobs.”
The team tested SCHEEPDOG using mammalian skin cells and epithelial cells from the lining of the kidney, which are often used to study cells’ collective movements. They found that the cells time-averaged signals generated along the two axes over a time window of about 20 seconds: Turning on the vertical electric field for 15 seconds and the horizontal field for 5 seconds, for instance, would cause the cells to migrate more in the vertical than in the horizontal direction.
“What the cells perceive is sort of a virtual angle, and that allows us to program any complex maneuver, like a full circle,” said Cohen. “That’s really surprising – that’s an amazing level of control that we wouldn’t have expected to be possible, especially with thousands of neighboring cells executing these maneuvers on command.”
The study “adds to the growing appreciation of cells’ responses to bioelectric aspects of their environment,” said Michael Levin, who directs the Center for Regenerative and Developmental Biology at Tufts University and was not involved in the research. “It demonstrates a technique to address not just individual cells’ activities in response to bioelectric cues, but the action of a cell collective, which is essential to understand how physical forces play into the kind of cooperativity we see in embryogenesis, regeneration and cancer.”
Using SCHEEPDOG, the team is expanding their studies to different cell types and contexts. Graduate student Gawoon Shim is investigating how varying levels of cell–cell adhesion impact directed cell migration – key information for eventual applications like regenerating skin, blood vessels and nerve cells in damaged tissue.
“This is the first step for whatever healing and regeneration we may need” in a variety of clinical contexts, said Shim, co-lead author of the study along with Zajdel. “We’re learning how to direct the cells where we need them, and then we can figure out what they’re going to do afterwards.”
Applying engineering principles to understand and control electrotaxis will deepen understanding of its role not only in cell movement, but also in growth and differentiation, said Cohen. While today’s cutting-edge tissue regeneration techniques usually involve pre-patterning new tissues, sculpting tissues with electric fields may allow for more flexibility and better outcomes. “In the long term, this might offer some very exciting, completely new ways of thinking about working with living tissues,” he said.
In addition to Zajdel, Shim and Cohen, other co-authors were Linus Wang, an undergraduate member of Princeton’s Class of 2021, and Alejandro Rossello-Martinez, who was a visiting master’s student from Imperial College London in spring 2019. The work was supported in part by the Princeton Catalysis Initiative.