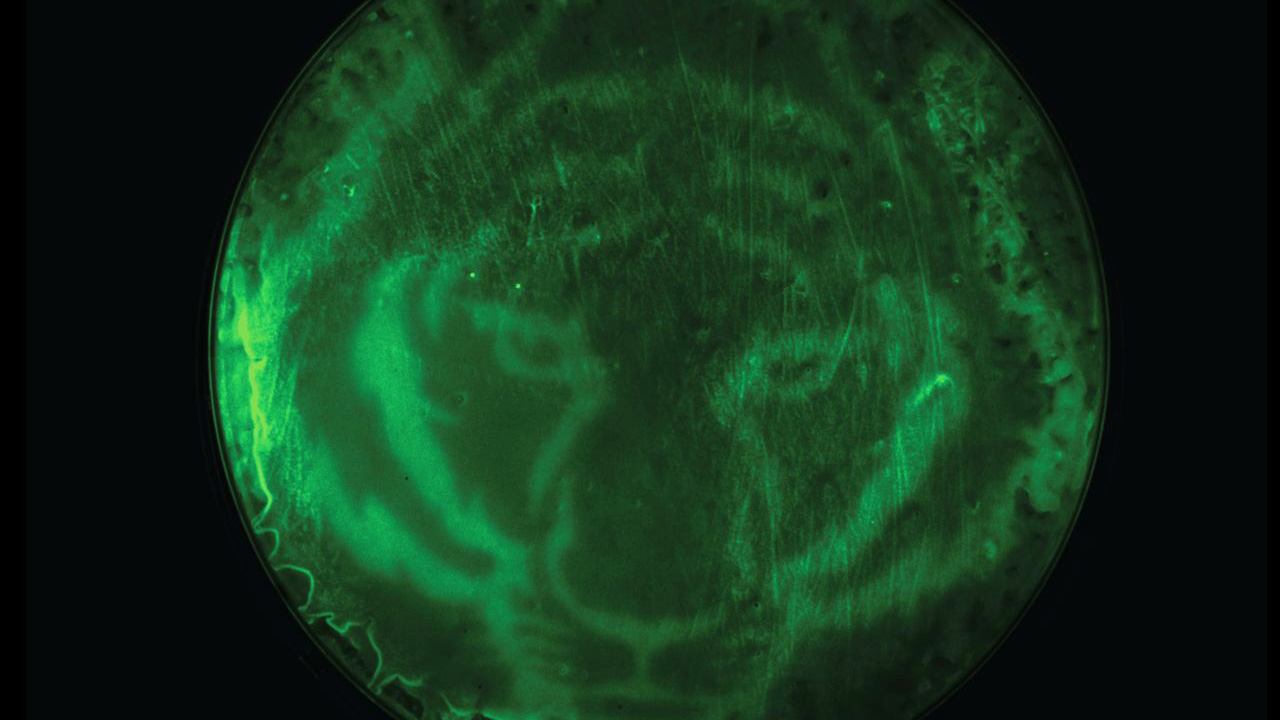
Flipping light on-off turns bacteria into chemical factories
By
on
“All you need is illumination,” said José Avalos, an assistant professor of chemical and biological engineering at Princeton University and at the Andlinger Center for Energy and the Environment, and senior author of the findings, published Sept. 7 in Nature Chemical Biology. “There are lots of potential benefits, one of them being the ability to easily tune and reverse the induction signal.”
The new work builds on Avalos and his colleagues’ previous research, described in Nature in 2018, in which they engineered yeast to produce chemicals in the presence or absence of light. E. coli, however, is even more widely used by scientists and engineers than yeast.
Avalos and his colleagues are not the first to create E. coli whose gene expression is controlled by light. But they are the first to use light to control production of chemicals. They also are the first to use light to control the lac operon, a group of genes that is most commonly utilized for chemical induction in E. coli. “The lac operon is the gold standard circuit that people have used for decades,” Avalos said. “It’s not an understatement to say that harnessing the lac operon is one of those key achievements that enabled the explosion in biotechnology.”
When scientists engineer E. coli to produce a protein or chemical through the lac operon, they typically make that function inducible rather than something that occurs all the time. This way, the bacteria culture can grow normally until scientists are ready to put it to use. Usually, researchers rely on adding a chemical to trigger the expression of the genetically engineered trait in question. But this method has some serious limitations. “If you add a chemical, that’s it, you’ve committed,” Avalos said. “It’s done and you cannot easily remove the chemical, so you just have to wait and see if you added the right dose.”
Instead of relying on a chemical inducer, Avalos and his colleagues’ newly engineered bacteria uses the absence of light to induce reactions that lead to chemical or protein production. This allows researchers to slow or stop the reaction by simply turning on the light. Light also allows them to control where the reaction occurs. In one demonstration, Avalos and his colleagues darkened only certain sections of their bacterial Petri dish with a stencil of a tiger, creating a fluorescent tiger print through the selectively activated bacteria’s reaction. “Again, that’s something you couldn’t easily do with a chemical, because you wouldn’t be able to control the diffusion of the chemical as easily,” Avalos said. Light, unlike chemicals, is also relatively inexpensive, he adds, so using it will reduce costs and probably the carbon footprint of processes.
OptoLac, Avalos and his colleagues’ new optogenetic—or light-based—method, now gives scientists the ability to harness the strength of preexisting lac operon technologies with added precision and control.
“The work was well-executed and adds a novel tool to the toolbox of optogenetic gene expression activators in E. coli,” said Mustafa Khammash, a professor of control theory and systems biology at ETH Zürich, who was not involved in the research. “Optogenetic gene expression offers the possibility to use light instead of small molecules to control a great variety of biological processes with minimal effort, and the authors illustrate this convincingly by demonstrating the use of OptoLAC to achieve impressive improvements in chemical and protein production in E. coli.”
E. coli is currently used for industrial production of a wide range of commodity and specialty chemicals, from building blocks of plastics and synthetic fibers, to high-end chemicals like pigments and fragrances. E. coli also is often used by scientists to better understand basic principles of metabolism, biosynthetic pathways and beyond. Therefore, this technology could have important implications not only in biotechnology, but also in basic research, Avalos said.
Avalos plans to explore further applications enabled by OptoLAC, including fine-tuning complex metabolic pathways, improving production of proteins that are difficult to make and controlling other interesting bacterial functions. “In a sense, a big part of our motivation was to break the mold of the way things are done now,” Avalos said. “One question we keep asking ourselves is, ‘How can we do this better?’”
The lead author of the Nature Chemical Biology paper is graduate student Makoto Lalwani of chemical and biological engineering. Co authors include undergraduates Samantha Ip of the Class of 2018 and Hinako Kawabe of the Class of 2019; Associate Research Scholar César Carrasco-López, molecular biology graduate student Catherine Day, and Evan Zhao who earned his Ph.D. in chemical and biological engineering in 2019.
The work was funded in part by the U.S. Department of Energy Office of Science, the National Science Foundation, the Pew Charitable Trusts, the Eric and Wendy Schmidt Transformative Technology Fund and the Camille Dreyfus Teacher-Scholar Award.