Princeton Engineering faculty members Hakan Türeci, Sarang Gopalakrishnan and Ran Raz are exploring new ways to achieve pristine quantum signals, track data in quantum systems, and define the theoretical capabilities of quantum computers.
Evading nature’s noise to reach pristine quantum signals
Moment by moment, our brains sift through a massive amount of sensory information to focus on what’s most pertinent. This ability to operate efficiently in noise-filled environments could inform the workings of quantum computers, according to Hakan Türeci, an associate professor of electrical and computer engineering.
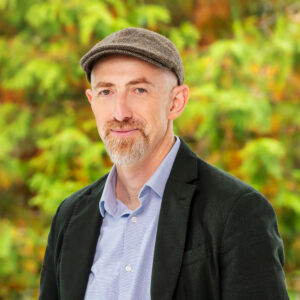
Trained as a theoretical physicist, Türeci is interested in the fundamental limits of computing with physical systems. He aims to help create a new generation of quantum computers that process information using minimal amounts of energy — and with negligible interference from the vagaries of ambient fields and other disturbances.
Türeci’s team is helping to build experimental quantum systems that use machine learning algorithms modeled after the brain’s neural processes, which are “designed for messy systems,” he said. These algorithms’ outputs may be less precise than the standards of digital computation, but this is often a more faithful reflection of the data they take in, he said.
At the same time, Türeci’s group is exploring methods to read qubits’ states more directly than current systems, which rely on several stages of amplifiers. With these techniques, the signals “are corrupted by a huge amount of noise, and we’ve been fighting this fact of life with the most basic tools,” said Türeci. “We can do a lot more to make these signals robust.”
Forensic approach shows gaps in the accounting for quantum data
Today’s computers keep track of massive amounts of data with relative ease. But for quantum computing, keeping track of data is still a central technological challenge. Sarang Gopalakrishnan studies the rules governing data in quantum systems, where the deep uncertainty of every exchange makes bookkeeping next to impossible. To get around that uncertainty, he’s developing new mathematical techniques to piece together events moment by moment.
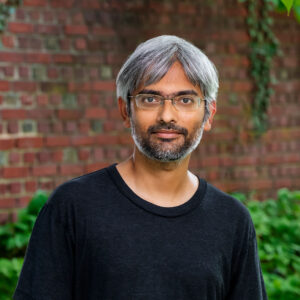
“I’m trying to exploit these connections between quantum information and what we know about how quantum systems evolve in time,” said Gopalakrishnan, assistant professor of electrical and computer engineering, who joined the Princeton faculty in September 2022. He said quantum systems are rich in answers to some of the deepest questions we can think to ask — you just have to know where to look.
His work touches on a fascinating mix of topics from information theory, computer science, machine learning, and signal processing. It also builds on the foundation laid by a Princeton icon: physicist and Nobel laureate Philip Anderson. Anderson pioneered modern solid-state physics and revolutionized the way scientists understand phases of matter. This more subtle view of matter turns out to be useful in accounting for quantum data. “In some sense, Anderson is the central figure in my entire field,” Gopalakrishnan said. “His work is central to all of my thinking.”
Theorist illuminates advantages of quantum computers
What problems can quantum computers approach that lie beyond the reach of classical computation? Ran Raz, a professor of computer science, is using mathematics to find out.
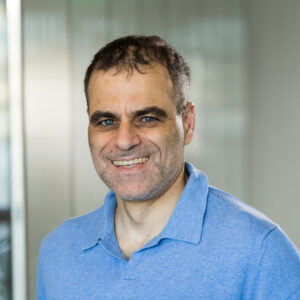
Evaluating the limits of computation can be extremely difficult. Computer scientists group problems into levels of complexity determined by their demand for resources, usually computation time or computer memory. One fundamental grouping begins with problems approachable by today’s classical computers and extends to powerful theoretical systems with enormous capabilities. Scientists refer to this ranking as the polynomial hierarchy.
In 2018, Raz and a former graduate student, Avishay Tal, demonstrated that quantum computers can grapple with problems that are outside the polynomial hierarchy. These problems cannot be solved, or have their answers checked, by classical computers, no matter how advanced.
Raz and Tal (now an assistant professor at the University of California-Berkeley) constructed their proof relative to an oracle — an imaginary answer key — and investigated how many times a quantum and a classical computer would need to consult the oracle to reach a solution. The quantum computer only needed one hint, while a classical computer could only solve the problem with an exponential number of hints.
“Our current understanding is that nature is governed by quantum physics, so as a theorist it’s interesting to consider the computational power of quantum physics — regardless of what types of quantum computers are built in the future,” said Raz.